Краткая история насекомых. Шестиногие хозяева планеты [заметки]
1
Тем, кто захочет познакомиться с палеонтологией поближе, можно порекомендовать две фундаментальные сводки, посвященные эволюции и палеонтологической летописи насекомых: Rasnitsyn A. P., Quicke D. L. J. (eds.) History of Insects. Dordrecht: Kluwer Academic Publishers, 2002; Grimaldi D., Engel M. S. Evolution of the insects. Cambridge: Cambridge University Press, 2005.
2
О различных аспектах тафономии (особенностей захоронения) насекомых см.: Жерихин В. В., Пономаренко А. Г., Расницын А. П. Введение в палеоэнтомологию. – М.: КМК, 2008.
3
Раньше она называлась Лабораторией членистоногих.
4
Довольно часто насекомых также находят в осадочных породах, образовавшихся на дне прибрежных морских лагун.
5
Насекомые известны примерно из 2500 местонахождений, но лишь 90 из них можно отнести к категории лагерштеттов. На эти 90 лагерштеттов приходится около 66 % всех описанных видов. Во многих других местонахождениях сохраняется лишь ограниченный спектр насекомых. Так, например, в грубозернистой породе порой можно найти одни только надкрылья жуков. См.: Schachat S. R., Labandeira C. C. Are Insects Heading Toward Their First Mass Extinction? Distinguishing Turnover From Crises in Their Fossil Record // Annals of the Entomological Society of America. 2020. Vol. XX. P. 1–20.
6
Hope F. W. Observations on Succinic Insects // Transactions of the Royal Entomological Society of London. 1837. Vol. 2. P. 46–57. В кавычках цитата из «Элегии на сельском кладбище» (1750) английского поэта Томаса Грея.
7
Seyfullah L. J. et al. Production and preservation of resins – past and present // Biological Reviews. 2018. Vol. 93. P. 1684–1714.
8
Whalley P., Jarzembowski E. A. A new assessment of Rhyniella, the earliest known insect, from the Devonian of Rhynie, Scotland // Nature. 1981. Vol. 291. P. 317.
9
Full R. J., Tu M. S. Mechanics of a rapid running insect: two-, four– and six-legged locomotion // Journal of Experimental Biology. 1991. Vol. 156. P. 215–231.
10
Bouček Z. The genera of chalcidoid wasps from Ficus fruit in the New World // Journal of Natural History. 1993. Vol. 27. P. 173–217.
11
Grenier J. K. et al. Evolution of the entire arthropod Hox gene set predated the origin and radiation of the onychophoran/arthropod clade // Current Biology. 1997. Vol. 7. P. 547–553.
12
Hughes C. L., Kaufman T. C. Hox genes and the evolution of the arthropod body plan // Evolution & development. 2002. Vol. 4. P. 459–499.
13
Павлов В. Я. Периодическая система членистых. – М.: ВНИРО, 2000.
14
Например, у губоногих многоножек из группы Geophilomorpha число пар ног (и, соответственно, сегментов) может варьировать от 87 до 177 (у вида Himantarium gabrielis), причем у самок, как правило, сегментов больше, чем у самцов.
15
Balbus S. A. Dynamical, biological and anthropic consequences of equal lunar and solar angular radii. Proceedings of the Royal Society A. 2014. Vol. 470: 20140263.
16
Libertín M. et al. Sporophytes of polysporangiate land plants from the early Silurian period may have been photosynthetically autonomous // Nature Plants. 2018. Vol. 4. P. 269–271.
17
Jeram A. J. et al. Land Animals in the Silurian: Arachnids and Myriapods from Shropshire, England // Science. 1990. Vol. 250. P. 658–661.
18
Brookfield M. E. et al. Myriapod divergence times differ between molecular clock and fossil evidence: U/Pb zircon ages of the earliest fossil millipede-bearing sediments and their significance // Historical Biology. 2021. Vol. 33. P. 2014–2018.
19
Hakbijl T. The Traditional, Historical and Prehistoric Use of Ashes as an Insecticide, with an Experimental Study on the Insecticidal Efficacy of Washed Ash // Environmental Archaeology. 2002. Vol. 7. P. 13–22.
20
Shear W. A. et al. Early Land Animals in North America: Evidence from Devonian Age Arthropods fromGilboa, New York // Science. 1984. Vol. 224. P. 492–494.
21
Carpenter F.M, Richardson E. S., Jr. Additional Insects in Pennsylvanian Concretions From Illinois // Psyche: A Journal of Entomology. 1971. Vol. 78. P. 267–295.
22
Clack J. A. et al. Phylogenetic and environmental context of a Tournaisian tetrapod fauna // Nature Ecology & Evolution. 2016. Vol. I: 0002.
23
См. полный список предполагаемых находок девонских насекомых: Haug C., Haug J. The presumed oldest flying insect: more likely a myriapod? // PeerJ. 2017. Vol. 5: e3402.
24
Prokop J. et al. Discovery of the oldest known Pterygota in the Lower Carboniferous of the Upper Silesian Basin in the Czech Republic (Insecta: Archaeorthoptera) // Geobios. 2008. Vol. 38. P. 383–387; Dvořák T. et al. New archaeorthopteran insects from the Carboniferous of Poland: Insights into tangled taxonomy // Acta Palaeontologica Polonica. 2019. Vol. 64. P. 787–796.
25
Schachat S. R. et al. Phanerozoic pO2 and the early evolution of terrestrial animals // Proceedings of the Royal Society B. 2018. Vol. 285: 20172631.
26
В умеренных широтах семенные растения стали преобладать уже во второй половине каменноугольного периода, когда на территории современной Сибири раскинулась кордаитовая «тайга», как называл ее отечественный палеоботаник Сергей Мейен. В тропических широтах Евроамерики в это время комплексы сухолюбивых семенных растений периодически прорывались в низины и отступали из них вслед за колебаниями климата. См.: DiMichele W.A. et al. Uplands, lowlands, and climate: Taphonomic megabiases and the apparent rise of a xeromorphic, drought-tolerant flora during the Pennsylvanian-Permian transition // Palaeogeography, Palaeoclimatology, Palaeoecology. 2020. Vol. 559: 109965.
27
DiMichele W. A., Aronson R. B. The Pennsylvanian-Permian vegetational transition: a terrestrial analogue to the onshore-offshore hypothesis // Evolution. 1992. Vol. 46. P. 807–824; Frederiksen N. O. The rise of the mesophytic flora // Geoscience and Man. 1972. Vol. 4. P. 17–28. Интересно, что хвойные растения, как и крылатые насекомые, тоже внезапно появились в середине каменноугольного периода в полностью готовом виде. Может быть, и те и другие пришли из общей прародины, располагавшейся где-то на возвышенностях?
28
Dudley R. et al. Gliding and the Functional Origins of Flight: Biomechanical Novelty or Necessity? // Annual Review of Ecology, Evolution, and Systematics. 2007. Vol. 38. P. 179–201.
29
Yanoviak S. P. et al. Gliding hexapods and the origins of insect aerial behaviour // Biology Letters. 2009. Vol. 5. P. 510–512.
30
Расницын А. П. Паранотальная теория происхождения крыльев насекомых: новая версия // Избранные труды по эволюционной биологии. – М.: КМК, 2005. C. 283–289; Prokop J. et al. Paleozoic Nymphal Wing Pads Support Dual Model of Insect Wing Origins // Current Biology. 2017. Vol. 27. P. 263–269.
31
Clark-Hachtel C. M. et al. Insights into insect wing origin provided by functional analysis of vestigial in the red flour beetle, Tribolium castaneum // Proceedings of the National Academy of Sciences. 2013. Vol. 110. P. 16951–16956.
32
Ортологами называются варианты одного и того же гена у разных эволюционных линий, унаследованные от общего предка и затем видоизменившиеся с течением времени.
33
Clark-Hachtel C. M., Tomoyasu Y. Two sets of candidate crustacean wing homologues and their implication for the origin of insect wings // Nature Ecology & Evolution. 2020. Vol. 4. P. 1694–1702.
34
Залесский Ю. М. Гигантские насекомые в пермских отложениях Приуралья // Природа. 1948. № 10. С. 82–84.
35
May M. L. Heat Exchange and Endothermy in Protodonata // Evolution. 1982. Vol. 36. P. 1051–1058; Dorrington G. E. Heavily loaded flight and limits to the maximum size of dragonflies (Anisoptera) and griffenflies (Meganisoptera) // Lethaia. 2016. Vol. 49. P. 261–274.
36
Надо отметить, что вентиляция трахей все-таки не является полностью пассивным процессом. Например, ученые выяснили, просвечивая синхротронным излучением жуков, муравьев и сверчков, что примерно каждые две секунды по стенкам их трахей пробегает волна сокращений. Трахейные стенки сдвигаются и раздвигаются, изменяя объем трахей примерно на 50 %. Для сравнения: в покое человеческое легкое вентилируется всего на 10 %, а при физической активности – на 75 %. Механизмы, которые стоят за этими сокращениями, не зависят от работы брюшной и летательной мускулатуры, т. е. представляют собой альтернативный способ подкачки воздуха. Westneat M. W. et al. Tracheal Respiration in Insects Visualized with Synchrotron X-ray Imaging // Science. 2003. Vol. 299. P. 558–560.
37
Klok C. J., Harrison J. F. Atmospheric Hypoxia Limits Selection for Large Body Size in Insects // PLoS ONE. 2009. Vol. 4: e3876.
38
Loudon C. Tracheal Hypertrophy in Mealworms: Design and Plasticity in Oxygen Supply Systems // Journal of Experimental Biology. 1989. Vol. 147. P. 217–235.
39
Kaiser A. et al. Increase in tracheal investment with beetle size supports hypothesis of oxygen limitation on insect gigantism // Proceedings of the National Academy of Sciences. 2007. Vol. 104. P. 13198–13203.
40
Dudley R. Atmospheric oxygen, giant Paleozoic insects and the evolution of aerial locomotor performance // Journal of Experimental Biology. 1998. Vol. 201. P. 1043–1050.
41
Цит. по: Четвериков С. С. Основной фактор эволюции насекомых // Проблемы общей биологии и генетики (воспоминания, статьи, лекции). – Новосибирск: Наука, 1983. – С. 232.
42
Picker M. et al. Influence of hindwing size in nemopterids (Insecta: Neuroptera: Nemopteridae) on predation by robber flies (Diptera: Asilidae) // Current Research in Neuropterology. Proceedings of the Fourth International Symposium on Neuropterology. BagnBres-de-Luchon, France, 1991. Canard, M., Aspiick, H. & Mansell, M.W. (Eds). Toulouse, France, 1992. P. 313–318.
43
Shear W. A., Kukalová-Peck J. The ecology of Paleozoic terrestrial arthropods: the fossil evidence // Canadian Journal of Zoology. 1990. Vol. 68. P. 1807–1834.
44
Правда, можно вспомнить об артроплеврах (Arthropleura) – гигантских палеозойских многоножках, которые достигали метра в длину. Но, может быть, от насекомоядных позвоночных их защищали токсины, подобные тем, что вырабатывают двупарноногие многоножки, к которым раньше относили артроплевр?
45
Dudley R. Atmospheric oxygen, giant Paleozoic insects and the evolution of aerial locomotor performance // Journal of Experimental Biology. 1998. Vol. 201. P. 1043–1050.
46
Clapham M. E., Karr J. A. Environmental and biotic controls on the evolutionary history of insect body size // Proceedings of the National Academy of Sciences. 2012. Vol. 109. P. 10927–10930.
47
Шаров А. Г. Филогения ортоптероидных насекомых. – М.: Наука, 1968.
48
Witton M. P. Pterosaurs: Natural History, Evolution, Anatomy. Princeton: Princeton University Press, 2013. P. 84–85.
49
Zhang Z. Q. Animal biodiversity: An introduction to higher-level classification and taxonomic richness // Zootaxa. 2011. Vol. 3148. P. 7–12.
50
Braddy S. J. et al. Giant claw reveals the largest ever arthropod // Biology letters. 2008. Vol. 4. P. 106–109.
51
Shulz A., Wagner T. Influence of forest type and tree species on canopy ants (Hymenoptera: Formicidae) in Budongo Forest, Uganda // Oecologia. 2002. Vol. 133. P. 224–232.
52
Четвериков С. С. Основной фактор эволюции насекомых // Проблемы общей биологии и генетики (воспоминания, статьи, лекции). Новосибирск: Наука, 1983. С. 232.
53
Polilov A. A. Small Is Beautiful: Features of the Smallest Insects and Limits to Miniaturization // Annual Review of Entomology. 2015. Vol. 60. P. 103–121.
54
Polilov A. A., Makarova A. S. The scaling and allometry of organ size associated with miniaturization in insects: A case study for Coleoptera and Hymenoptera // Scientific Reports. 2017. Vol. 7: 43095.
55
Huber J. T., Noyes J. S. A new genus and species of fairyfly, Tinkerbella nana (Hymenoptera, Mymaridae), with comments on its sister genus Kikiki, and discussion on small size limits in arthropods // Journal of Hymenoptera Research. 2013. Vol. 32. P. 17–44.
56
Maraun et al. Awesome or ordinary? Global diversity patterns of oribatid mite // Ecography. 2007. Vol. 30. P. 209–216.
57
Zhang Y. et al. Flight Performance of the Soybean Aphid, Aphis glycines (Hemiptera: Aphididae) Under Different Temperature and Humidity Regimens // Environmental Entomology. 2008. Vol. 37. P. 301–306.
58
Hu G. et al. Mass seasonal bioflows of high-flying insect migrants // Science. 2016. Vol. 354. P. 1584–1587.
59
Liebherr J. K. The Mecyclothorax beetles (Coleoptera, Carabidae, Moriomorphini) of Haleakala, Maui: Keystone of a hyperdiverse Hawaiian radiation // Zookeys. 2015. Vol. 544. P. 1–407.
60
Lapoint R. T. et al. Phylogenetic relationships in the spoon tarsus subgroup of Hawaiian drosophila: Conflict and concordance between gene trees // Molecular Phylogenetics and Evolution. 2011. Vol. 58. P. 492–501.
61
Yamamoto S. et al. Evolutionary stasis in enigmatic jacobsoniid beetles // Gondwana Research. 2017. Vol. 45. P. 275–281.
62
Cai C., Huang D. Omma daxishanense sp. nov., a fossil representative of an extant Australian endemic genus recorded from the Late Jurassic of China (Coleoptera: Ommatidae) // Alcheringa. 2017. Vol. 41. P. 277–283.
63
Точнее говоря, в ископаемом состоянии был известен не сам ныне живущий род Latimeria, а отряд целакантообразных рыб, к которому он принадлежит.
64
Sinclair B. J., Kirk-Spriggs A. H. Alavesia Waters and Arillo – a Cretaceous‐era genus discovered extant on the Brandberg Massif, Namibia (Diptera: Atelestidae) // Systematic Entomology. 2010. Vol. 35. P. 268–276.
65
Первый богомолопалочник из балтийского янтаря стал достоянием научной общественности в 1997 г., а статья с описанием ныне живущих представителей этой группы, пойманных в Африке, появилась в 2002 г. Богомолопалочников сразу отнесли к новому отряду. Это стало сенсацией, ведь открыть ранее неизвестный отряд ныне живущих насекомых – почти то же самое, что открыть новую планету Солнечной системы; последний раз такое произошло в 1914 г., когда в канадских горах энтомологи поймали тараканосверчков (Grylloblattodea). Но с тех пор страсти поулеглись, и многие считают, что богомолопалочники – это просто отдельная группа в рамках более крупного отряда, куда входят также тараканосверчки вместе со своей многочисленной вымершей родней. См.: Arillo A., Engel M. S. Rock Crawlers in Baltic Amber (Notoptera: Mantophasmatodea) // American Museum Novitates. 2006. V. 3539. P. 1–10.
66
Yan E. V. et al. The most mysterious beetles: Jurassic Jurodidae (Insecta: Coleoptera) from China // Gondwana Research. 2014. Vol. 25. P. 214–225.
67
Fikáček et al. A long-living species of the hydrophiloid beetles: Helophorus sibiricus from the early Miocene deposits of Kartashevo (Siberia, Russia) // Zookeys. 2011. Vol. 130. P. 239–254.
68
Hörnschemeyer T. et al. How long can insect species exist? Evidence from extant and fossil Micromalthus beetles (Insecta: Coleoptera). Zoological Journal of the Linnean Society. 2010. Vol. 158. P. 300–311.
69
Benton M. When Life Nearly Died: The Greatest Mass Extinction of All Time. L.: Thames & Hudson, 2003.
70
Волков А. Н. Жуки из межтрапповых отложений Тунгусского бассейна // Современная палеонтология: классические и новейшие методы. Тезисы докладов. – М.: ПИН РАН, 2013. – С. 12–13.
71
Nicholson et al. Changes to the Fossil Record of Insects through Fifteen Years of Discovery // PLoS ONE. 2015. Vol. 10: e0128554.
72
Sallan L., Galimberti A. K. Body-size reduction in vertebrates following the end-Devonian mass extinction // Science. 2015. Vol. 350. P. 812–815.
73
Botha-Brink et al. Breeding Young as a Survival Strategy during Earth’s Greatest Mass Extinction // Scientific Reports. 2016. Vol. 6: 24053.
74
Расницын А. П. Когда жизнь и не думала умирать // Природа, 2012. № 9. С. 39–48.
75
Troast D. et al. A Global Population Genetic Study of Pantala flavescens. PLoS ONE. 2016. Vol. 11: e0148949.
76
Zheng D. et al. The discovery of an Early Cretaceous dragonfly Hemeroscopus baissicus Pritykina, 1977 (Hemeroscopidae) in Jiuquan, Northwest China, and its stratigraphic implications // Cretaceous Research. 2015. Vol. 52. P. 316–322.
77
Mayhew P. J. Why are there so many insect species? Perspectives from fossils and phylogenies // Biological Reviews. 2007. Vol. 82. P. 425–454.
78
Coope G. R. Several million years of stability among insect species because of, or in spite of, Ice Age climatic instability? // Philosophical Transactions of the Royal Society B. 2004. V. 359. P. 209–214.
79
Coope G. R., Wilkins A. S. The Response of Insect Faunas to Glacial-Interglacial Climatic Fluctuations // Philosophical Transactions of the Royal Society B. 1994. Vol. 344. P. 19–26.
80
Archibald B., Farrell B. D. Wheeler’s dilemma // Acta Zoologica Cracoviensia. 2003. Vol. 46. P. 17–23.
81
Василий Великий. Св. Беседы на Шестоднев. М.: Издательство Московского подворья Свято-Троицкой Сергиевой лавры, 1999. С. 236.
82
Merian M. S. Metamorphosis Insectorum Surinamensium. Amsterdam: Voor den auteur, als ook by G. Valck, 1705.
83
Swammerdam J. Bybel der natuure. V. 1, 2. Leyden: I. Severinus, 1737, 1738.
84
Nicholson D. B. et al. Fossil evidence for key innovations in the evolution of insect diversity // Philosophical Transactions of the Royal Society B. 2014. Vol. 281: 20141823.
85
Algeo T. J., Scheckler S. E. Terrestrial-marine teleconnections in the Devonian: links between the evolution of land plants, weathering processes, and marine anoxic events // Philosophical Transactions of the Royal Society B. 1998. Vol. 353. P. 113–130.
86
Nel A. et al. The earliest holometabolous insect from the Carboniferous: a “crucial” innovation with delayed success (Insecta Protomeropina Protomeropidae) // Annales de la Société entomologique de France. 2007. Vol. 43. P. 349–355. На звание древнейших насекомых с полным превращением также претендует вымерший отряд Miopmoptera, встречающийся с середины каменноугольного периода, но о его систематическом положении идут споры.
87
Truman J. W., Riddiford L. M. The evolution of insect metamorphosis: a developmental and endocrine view // Philosophical Transactions of the Royal Society B. 2019. Vol. 374: 20190070
88
Соответственно, если верна гипотеза Берлезе, то термины «личинка» и «нимфа» не эквивалентны. Первый из них можно применять только к голометаболам (например, личинка мухи), а второй – только к насекомым с неполным превращением (например, нимфа стрекозы).
89
Truman J.W., Riddiford L. M. The origins of insect metamorphosis // Nature. 1999. Vol. 401. P. 447–452.
90
Mitscherling J., Horchler P. J. Spatial patterns of folivory at Acer pseudoplatanus L. in the Leipzig forest canopy // The Canopy of a Temperate Floodplain Forest / Unterseher M. et al (eds). Leipzig, 2007. P. 52–56.
91
Smith D. M., Nufio C. R. Levels of Herbivory in Two Costa Rican Rain Forests: Implications for Studies of Fossil Herbivory // Biotropica. 2004. Vol. 36. P. 318–326.
92
Rinker H. B., Lowman M. D. Insect Herbivory in Tropical Forests // Forest Canopies. 2nd edn / Lowman M. D., Rinker H. B. (ed). Burlington: Elsevier Academic Press, 2004. P. 359–386
93
Iannuzzi R., Labandeira C. The Oldest Record of External Foliage Feeding and the Expansion of Insect Folivory on Land // Annals of the Entomological Society of America. 2008. Vol. 101. P. 79–94.
94
Labandeira C. C., Allen E. G. Minimal insect herbivory for the Lower Permian Coprolite Bone Bed site of north-central Texas, USA, and comparison to other Late Paleozoic floras // Palaeogeography, Palaeoclimatology, Palaeoecology. 2007. Vol. 247. P. 197–219.
95
Edwards D., Selden P. A. The Development of Early Terrestrial Ecosystems // Botanical Journal of Scotland. 1992. P. 337–366.
96
Plantinga A. Where the Conflict Really Lies: Science, Religion, and Naturalism. New York: Oxford University Press, 2011. P. 107.
97
Мартынов А. В. О двух типах крыльев насекомых и их эволюции // Русский зоологический журнал. 1924. Т. 4. С. 157–185.
98
Busch A. et al. Functional diversification of horizontally acquired glycoside hydrolase family 45 (GH45) proteins in Phytophaga beetles // BMC Evolutionary Biology. 2019. Vol. 19: 100; Kirsch R. et al. Horizontal gene transfer and functional diversification of plant cell wall degrading polygalacturonases: Key events in the evolution of herbivory in beetle // Insect Biochemistry and Molecular Biology. 2014. Vol. 52. P. 33–50.
99
McKenna D. D. et al. The evolution and genomic basis of beetle diversity // Proceedings of the National Academy of Sciences. 2019. Vol. 116. P. 24729–24737.
100
Shelomi M. et al. Horizontal Gene Transfer of Pectinases from Bacteria Preceded the Diversification of Stick and Leaf Insects // Scientific Reports. 2016. Vol. 6: 26388.
101
Salem H. Drastic Genome Reduction in an Herbivore’s Pectinolytic Symbiont // Cell. 2017. Vol. 171. P. 1520–1531.
102
Anbutsu H. et al. Small genome symbiont underlies cuticle hardness in beetles // Proceedings of the National Academy of Sciences. 2017. Vol. 114. E8382–E8391.
103
Карл Э. Очень голодная гусеница. – М.: Розовый жираф, 2019.
104
Dobler S. et al. Community-wide convergent evolution in insect adaptation to toxic cardenolides by substitutions in the Na, K-ATPase // Proceedings of the National Academy of Sciences. 2012. Vol. 109. P. 13040–13045.
105
Wheat C. W. et al. The genetic basis of a plant – insect coevolutionary key innovation // Proceedings of the National Academy of Sciences. 2007. Vol. 104. P. 20427–20431.
106
Ehrlich P. R., Raven P. H. Butterflies and Plants: A Study in Coevolution // Evolution. 1964. Vol. 18. P. 586–608.
107
Pauly P. J. Fighting the Hessian fly: American and British Responses to insect invasion, 1776–1789 // Environmental History. 2002. Vol. 7. P. 377–400.
108
Morton P. K., Schemerhorn B. J. Population Structure and the Colonization Route of One of the Oldest North American Invasive Insects: Stories from the Worn Road of the Hessian Fly, Mayetiola destructor (Say) // PLoS ONE. 2013. Vol. 8: e59833.
109
Takasuka K. et al. Host manipulation by an ichneumonid spider ectoparasitoid that takes advantage of preprogrammed web-building behaviour for its cocoon protection // Journal of Experimental Biology. 2015. Vol. 218. P. 2326–2332.
110
Andreas P. et al. Cytokinins Are Abundant and Widespread among Insect Species // Plants. 2020. Vol. 9: 208.
111
Tanaka Y. et al. Phytohormones in Japanese Mugwort Gall Induction by a Gall-Inducing Gall Midge // Bioscience, Biotechnology, and Biochemistry. 2013. Vol. 77. P. 1942–1948.
112
Bartlett L., Connor E. F. Exogenous phytohormones and the induction of plant galls by insects // Arthropod-Plant Interactions. 2014. Vol. 8. P. 339–348.
113
Schönrogge K. et al. The protein content of tissues in cynipid galls (Hymenoptera: Cynipidae): Similarities between cynipid galls and seeds // Plant, Cell and Environment. 2000. Vol. 23. P. 215–222.
114
Allison S. D., Schultz J. C. Biochemical responses of chestnut oak to a galling cynipid // Journal of Chemical Ecology. 2005. Vol. 31. P. 151–166.
115
Larew H. G. Oak galls preserved by the eruption of mount vesuvius in A.D. 79, and their probable use // Economic Botany. 1987. Vol. 41. P. 33–40.
116
Scott A.C. et al. The fossil record of leaves with galls // Plant galls / Williams M. A.J. (ed.) Oxford: Clarendon Press, 1994. P. 447–470.
117
Opler P. A. Fossil Lepidopterous Leaf Mines Demonstrate the Age of Some Insect-Plant Relationships // Science. 1973. Vol. 179. P. 1321–1323.
118
Leckey E. H., Smith D. M. Host fidelity over geologic time: restricted use of oaks by oak gallwasps // Journal of Paleontology. 2015. Vol. 89. P. 236–244.
119
Grissell E. E. Hymenopteran Biodiversity: Some Alien Notions // American Entomologist. 1999. Vol. 45. P. 235–244.
120
Eggleton P., Belshaw R. Insect Parasitoids: An Evolutionary Overview // Philosophical Transactions of The Royal Society B. 1992. Vol. 337. P. 1–20.
121
Дарвин Ч. Избранные письма. М.: Изд-во иностранной литературы, 1950. С. 130.
122
Smith M.A. et al. Extreme diversity of tropical parasitoid wasps exposed by iterative integration of natural history, DNA barcoding, morphology, and collections // Proceedings of the National Academy of Sciences. 2008. Vol. 105. P. 12359–12364.
123
Baur H. et al. Morphometric analysis and taxonomic revision of Anisopteromalus Ruschka (Hymenoptera: Chalcidoidea: Pteromalidae) – an integrative approach // Systematic Entomology. 2014. Vol. 39. P. 691–709.
124
Owen J. et al. Species diversity of Ichneurnonidae and Serphidae (Hymenoptera) in an English suburban garden // Biological Journal of the Linnean Society. 1981. Vol. 16. P. 315–336.
125
Grissell E. E. Hymenopteran Biodiversity: Some Alien Notions // American Entomologist. 1999. Vol. 45. P. 235–244.
126
Wang Z. et al. Parasitoid wasps as effective biological control agents // Journal of Integrative Agriculture. 2019. Vol. 18. P. 705–715.
127
Pimentel D. Natural Control of Caterpillar Populations on Cole Crops // Journal of Economic Entomology. 1961. Vol. 54. P. 889–892.
128
Sanders D. et al. Trophic assimilation efficiency markedly increases at higher trophic levels in four-level host – parasitoid food chain // Philosophical Transactions of The Royal Society B. 2016. Vol. 283: 20153043.
129
Condon M. A. Lethal interactions between parasites and prey increase niche diversity in a tropical community // Science. 2014. Vol. 343. P. 1240–1244.
130
Ronquist F. Evolution of parasitism among closely related species: phylogenetic relationships and the origin of inquilinism in gall wasps (Hymenoptera, Cynipidae) // Evolution. 1994. Vol. 48. P. 241–266.
131
Vilhelmsen L., Turrisi G. F. Per arborem ad astra: Morphological adaptations to exploiting the woody habitat in the early evolution of Hymenoptera // Arthropod Structure & Development. 2011. Vol. 40. P. 2–20.
132
Жерихин В. В. Исторические изменения разнообразия насекомых // Избранные труды по палеоэкологии и филоценогенетике. – М.: КМК, 2003. – C. 321–329.
133
Augusto L. et al. The enigma of the rise of angiosperms: can we untie the knot? // Ecology Letters. 2014. Vol. 17. P. 1326–1338.
134
Lopes A. J. O. et al. Parasitism by Ixodiphagus Wasps (Hymenoptera: Encyrtidae) in Rhipicephalus sanguineus and Amblyomma Ticks (Acari: Ixodidae) in Three Regions of Brazil // Journal of Economic Entomology. 2012. Vol. 105. P. 1979–1981.
135
Thomas van de Kamp et al. Parasitoid biology preserved in mineralized fossils // Nature Communications. Vol. 9: 3325.
136
Lohrmann V., Engel M. S. The wasp larva’s last supper: 100 million years of evolutionary stasis in the larval development of rhopalosomatid wasps (Hymenoptera: Rhopalosomatidae) // Fossil record. 2017. Vol. 20. P. 239–244.
137
Bézier A. et al. Polydnaviruses of Braconid Wasps Derive from an Ancestral Nudivirus // Science. 2009. V. 323. P. 926–930.
138
Coffman K. A. et al. A mutualistic poxvirus exhibits convergent evolution with other heritable viruses in parasitoid wasps // Journal of Virology. 2020. Vol. 94: e02059–19.
139
Dheilly N. M. et al. Who is the puppet master? Replication of a parasitic wasp-associated virus correlates with host behaviour manipulation. Proceedings of the Royal Society B. 2015. Vol. 282: 20142773.
140
Jacas J. A. et al. Virus-like particles in the poison gland of the parasitic wasp Opius concolor // Annals of Applied Biology. 1997. Vol. 130. P. 587–592.
141
Zuk M. et al. Silent night: adaptive disappearance of a sexual signal in a parasitized population of field cricket. Biology Letters. 2006. Vol. 2. P. 521–524.
142
Feener D. H., Brown B. V. Diptera as parasitoids // Annual Review of Entomology. 1997. Vol. 42. P. 73–97.
143
Poinar G. Jr. Stenaspidiotus microptilus n. gen., n. sp. (Coleoptera: Chrysomelidae: Chrysomelinae) in Dominican amber, with evidence of tachinid (Diptera: Tachinidae) oviposition // Historical Biology. 2013. Vol. 25. P. 101–105.
144
Yeates D. K., Greathead D. The evolutionary pattern of host use in the Bombyliidae (Diptera): a diverse family of parasitoid flies // Biological Journal of the Linnean Society. 1997. Vol. 60. P. 149–185.
145
Kerr P. H., Winteron S. L. Do parasitic flies attack mites? Evidence in Baltic amber // Biological Journal of the Linnean Society. 2008. Vol. 93. P. 9–13.
146
Belshaw R. Life history characteristics of Tachinidae (Diptera) and their effect on polyphagy // Parasitoid community ecology / Hawkins B. A., Sheehan W. (eds) Oxford: Oxford University Press, 1994. P. 145–162.
147
Впрочем, были и каллиграмматиды, лишенные глазчатых пятен, – как мы знаем, они есть и далеко не у всех бабочек.
148
De Bona S. et al. Predator mimicry, not conspicuousness, explains the efficacy of butterfly eyespots // Proceedings of the Royal Society B. 2015. Vol. 282: 20150202.
149
Labandeira C. C. et al. The evolutionary convergence of mid-Mesozoic lacewings and Cenozoic butterflies // Proceedings of the Royal Society. 2016. Vol. 283: 20152893.
150
Ren D. et al. A probable pollination mode before angiosperms: Eurasian, long-proboscid scorpionflies // Science. 2009. Vol. 326: 840–847.
151
Friis E. M. et al. Diversity in obscurity: fossil flowers and the early history of angiosperms // Philosophical Transactions of the Royal Society B. 2010. Vol. 365. P. 369–382.
152
Friis E. M. Actinocalyx gen. nov., sympetalous angiosperm flowers from the Upper Cretaceous of southern Sweden // Review of Palaeobotany and Palynology. 1985. Vol. 45. P. 171–83.
153
Swinscow T. D. V. Friedrich Welwitsch, 1806–72: A centennial memoir // Biological Journal of the Linnean Society. 1972. Vol. 4. P. 269–289.
154
Насекомоопыление характерно и для некоторых современных саговников (после хвойных второй по числу видов отдел голосеменных растений), однако у них наградой для опылителей служат пыльца и мякоть шишек, а не опылительные капли.
155
Bolinder K. et al. From near extinction to diversification by means of a shift in pollination mechanism in the gymnosperm relict Ephedra (Ephedraceae, Gnetales) // Botanical Journal of the Linnean Society. 2016. Vol. 180. P. 461–477.
156
Khramov A. V., Lukashevich E. D. A Jurassic dipteran pollinator with an extremely long proboscis // Gondwana Research. 2019. Vol. 71. P. 210–215.
157
Peñalver E. et al. Long-Proboscid Flies as Pollinators of Cretaceous Gymnosperms // Current Biology. 2015. Vol. 25. P. 1917–1923.
158
Friedman W. E. The meaning of Darwin’s “abominable mystery” // American Journal of Botany. 2009. Vol. 96. P. 5–21.
159
Ollerton J. et a. How many flowering plants are pollinated by animals? // Oikos. 2011. Vol. 120. P. 321–326.
160
Уоллес А. Тропическая природа. М.: Мысль, 1975. С. 42.
161
Pokorny T. et al. Dispersal ability of male orchid bees and direct evidence for long-range flights // Apidologie. 2015. Vol. 46. P. 224–237.
162
Hodges S. A. Floral Nectar Spurs and Diversification // International Journal of Plant Sciences. 1997. Vol. 158. P. S81–S88.
163
Существует множество исследований, посвященных влиянию периодической смены опылителей на радиацию орхидных. Вот только одно из них: Johnson S. D. et al. Phylogeny and radiation of pollination systems in Disa (Orchidaceae) // American Journal of Botany. 1998. Vol. 85. P. 402–411.
164
Храмов А. В. и др. Длиннохоботковые насекомые-нектарофаги в палеонтологической летописи // Энтомологическое обозрение, 2020. Т. 99. С. 737–844.
165
Голенкин М. И. Победители в борьбе за существование. Исследование причин и условий завоевания Земли покрытосеменными растениями в середине мелового периода. – М.: Изд-во Ассоциации научно-исследовательских институтов при физико-математическом факультете 1-го МГУ, 1927.
166
Bond W. J. The tortoise and the hare: ecology of angiosperm dominance and gymnosperm persistence // Biological Journal of the Linnean Society. 1989. Vol. 36. P. 227–249.
167
Boyce C. K. et al. Angiosperm leaf vein evolution was physiologically and environmentally transformative // Proceedings of the Royal Society B. 2009. Vol. 276. P. 1771–1776.
168
Feild T.S. et al. Fossil evidence for Cretaceous escalation in angiosperm leaf vein evolution // Proceedings of the National Academy of Sciences. 2011. Vol. 108. P. 8363–8366.
169
Peris D. et al. False Blister Beetles and the Expansion of Gymnosperm-Insect Pollination Modes before Angiosperm Dominance // Current Biology. 2017. Vol. 27. P. 897–904.
170
Rust J. Fossil record of mass moth migration // Nature. 2000. Vol. 405. P. 530–531.
171
Heine E. M. Observations on the Pollination of New Zealand Flowering Plants // Transactions and Proceedings of the Royal Society of New Zealand. 1938. Vol. 67. P. 133–148.
172
Godley E. J. Flower biology in New Zealand // New Zealand Journal of Botany. 1979.Vol. 17: 441–466.
173
Ollerton J. Pollinator Diversity: Distribution, Ecological Function, and Conservation // Annual Review of Ecology, Evolution, and Systematics. 2017. Vol. 48. P. 353–376.
174
Engel M. S. et al. A Honeybee from the Miocene of Nevada and the Biogeography of Apis (Hymenoptera: Apidae: Apini) // Proceedings of the California Academy of Sciences, Series 4. 2009.Vol. 60. P. 23–38.
175
Wood C. S. New Evidence for a Late Introduction of Malaria into the New World // Current Anthropology. 1975. Vol. 16. P. 93–96.
176
Высказывалось предположение, что еще в палеозое кровососанием могли заниматься некоторые диафаноптеры (Diaphanopterodea) небольшого размера, внешне похожие на комаров. Теоретически ничто не мешало этим родичам палеодиктиоптер использовать свой колющий хоботок для прокалывания шкур каких-нибудь звероящеров, но проверить эту гипотезу, скорее всего, не удастся никогда. См.: Shear W. A., Kukalová-Peck J. The ecology of Paleozoic terrestrial arthropods: the fossil evidence // Canadian Journal of Zoology. 1990. Vol. 68. P. 1807–1834.
177
Wiegmann B. M. et al. Episodic radiations in the fly tree of life // Proceedings of the National Academy of Sciences. 2011. Vol. 108. P. 5690–5695. Возможно, эта цифра несколько завышена, но уж пять-семь раз кровососание возникало у двукрылых совершенно точно. Считайте сами: среди длинноусых двукрылых оно возникло один раз у москитов (подсемейство бабочниц Psychodidae) и один-два раза в подотряде Culicomorpha, к которому относятся комары, мошки, мокрецы и их родня. Среди мух кровососание один-два раза появилось в инфраотряде Tabanomorpha (мухи-бекасницы и слепни), один раз – в надсемействе Hippoboscoidea, включающем цеце и мух-кровососок, и один раз – в семействе Muscidae, к которому относятся мухи-жигалки.
178
Стоит отметить, что первые кровососы среди двукрылых могли появиться уже в юрском периоде. Например, в средней юре Китая были найдены мухи-бекасницы Elliprhagio с удлиненным хоботком. По одной версии, они были кровососущими, по другой – питались сладковатыми выделениями растений. В юре существовали и мошки (Simuliidae), правда, их находки ограничиваются одной-единственной куколкой Simulimima. Кроме того, надо иметь в виду, что насекомоносные янтари известны только с раннего мела, а до этого мелкие кровососы вроде мокрецов и москитов имели немного шансов попасть в палеонтологическую летопись.
179
Но для каких-то групп нельзя исключать и прямо противоположную последовательность событий: благодаря тому, что во взрослом возрасте двукрылые стали пить кровь, на личиночной стадии они смогли заселить скудные местообитания, как это сделали мошки (Simulidae), личинки которых живут в чистой (а значит, бедной органикой) проточной воде.
180
Van Riper C. et al. The Epizootiology and Ecological Significance of Malaria in Hawaiian Land Birds // Ecological Monographs. 1986. Vol. 56(4). P. 327–344.
181
Poinar G., Telford S. R. Paleohaemoproteus burmacis gen. n., sp. n. (Haemospororida: Plasmodiidae) from an Early Cretaceous biting midge (Diptera: Ceratopogonidae) // Parasitology. 2005. Vol. 131. P. 79–84.
182
Poinar G. What Fossils Reveal About the Protozoa Progenitors, Geographic Provinces, and Early Hosts of Malarial Organisms // American Entomologist. 2016. Vol. 62. P. 22–25.
183
Правда, скептики указывают, что количество булавовидных сенсилл показательно только в случае кровососов, прибегающих к активному поиску добычи. Засадным кровососам, которые дожидаются, пока мимо пройдет какое-то позвоночное, большое число сенсилл на щупиках не нужно, даже если они пьют кровь мелких животных.
184
Greenwalt D. E. et al. Hemoglobin-derived porphyrins preserved in a Middle Eocene blood-engorged mosquito // Proceedings of the National Academy of Sciences. 2013. Vol. 110. P. 18496–18500.
185
Poinar G. O., Hess R. Ultrastructure of 40-Million-Year-Old Insect Tissue // Science. 1982. Vol. 215. P. 1241–1242.
186
Jones E. D. Ancient DNA: a history of the science before Jurassic Park // Studies in History and Philosophy of Science Part C. 2018. Vol. 68–69. P. 1–14.
187
Jones E. D. Ancient DNA: a history of the science before Jurassic Park // Studies in History and Philosophy of Biol & Biomed Sci. 2018. Vol. 68–69. P. 1–14.
188
Cano R. J. et al. Amplification and sequencing of DNA from a 120–135-million-year-old weevil // Nature. 1993. Vol. 363. P. 536–538.
189
Gutiérrez G., Marín A. The Most Ancient DNA Recovered from an Amber-Preserved Specimen May Not Be as Ancient as It Seems // Molecular Biology and Evolution. 1998. Vol. 15. P. 926–929.
190
Austin J. J. et al. Problems of reproducibility-does geologically ancient DNA survive in amber-preserved insects? // Proceedings of the Royal Society of London B. 1997. Vol. 264. P. 467–474.
191
Penney D. et al. Absence of Ancient DNA in Sub-Fossil Insect Inclusions Preserved in ‘Anthropocene’ Colombian Copal // PLoS ONE. 2013. Vol. 8: e73150.
192
Cano R. J., Borucki M. K. Revival and identification of bacterial spores in 25-to 40-million-year-old Dominican amber // Science. 1995. Vol. 268. P. 1060–1064.
193
Greenblatt C. L. et al. Micrococcus luteus – survival in amber // Microbial ecology. 2004. Vol. 48. P. 120–127.
194
Willerslev E. et al. Long-Term persistence of bacterial DNA // Current Biology. 2004. Vol. 14: R9–R10.
195
Law R. Wheeled Transport in Pre-Colonial West Africa // Journal of the International African Institute. 1980. Vol. 50. P. 249–262.
196
McPhee A. The Economic Revolution in British West Africa. L.: Routledge, 1926.
197
Law R. A West African Cavalry State: The Kingdom of Oyо // Journal of African History. 1975. Vol. 16. P. 1–15.
198
Alsan M. The Effect of the TseTse Fly on African Development // American Economic Review. 2015. V. 105. P. 382–410.
199
Nash T. A. Africa’s Bane: The Tsetse Fly. London: Collins, 1969. P. 31.
200
Там же.
201
Tailor D. B. et al. Economic Impact of Stable Flies (Diptera: Muscidae) on Dairy and Beef Cattle Production // Journal of Medical Entomology. 2012. V. 49. P. 198–209.
202
Точно так же устроено размножение и мух-кровососок (Hippoboscoidea), которые рождают на свет готовую личинку последнего возраста. Вместе с мухами цеце они являют собой предельный случай эволюционного тренда, характерного для кровососущих двукрылых, когда имаго перекладывает на себя заботу о пропитании, снимая ее с личинки. У большинства других насекомых ситуация обратная: питается в основном личинка, а взрослые особи иногда могут вовсе не утруждать себя поиском пищи и жить за счет питательных веществ, накопленных на личиночной стадии, как это наблюдается у поденок и некоторых комаров.
203
Hargrove J. W. The flight performance of tsetse flies // Journal of Insect Physiology. 1975. P. 1385–1395.
204
Wingate P. Rain Doctor. London: Heinemann, 1958. P. 73.
205
Wedmann et al. Fossil insects from the Late Oligocene Enspel Lagerstätte and their palaeobiogeographic and palaeoclimatic significance. Palaeobiodiversity and Palaeoenvironments. 2010. Vol. 90. P. 49–58.
206
Cockerell T. D. A. A Fossil Tsetse-fly in Colorado. Nature. 1907. Vol. 76. P. 414.
207
Pamuk S. The Black Death and the origins of the ‘Great Divergence’ across Europe, 1300–1600 // European Review of Economic History. 2007. Vol. 11. P. 289–317.
208
Whiting M. F. et al. A molecular phylogeny of fleas (Insecta: Siphonaptera): origins and host associations // Cladistics. 2008. Vol. 24. P. 677–707.
209
Liu S., Hua B. Ultramorphology of the proventriculus in Panorpidae and Bittacidae (Mecoptera) // Micron. 2009. Vol. 40. P. 899–905.
210
Список находок ископаемых блох см.: Perrichot V. et al. First extinct genus of a flea (Siphonaptera: Pulicidae) in Miocene amber from the Dominican Republic // Zootaxa. 2012. Vol. 3438. P. 54–61.
211
Gao T. et al. Mid-Mesozoic Flea-like Ectoparasites of Feathered or Haired Vertebrates // Current Biology. 2012. Vol. 22. P. 732–735.
212
Gao T. et al. The first flea with fully distended abdomen from the Early Cretaceous of China // BMC Evolutionary Biology. 2014. Vol. 14: 168.
213
Расницын А. П., Стрельникова О. Д. Пищеварительная система раннемелового ископаемого Saurophthirus longipes Ponomarenko (Insecta,? Aphaniptera, Saurophthiroidea) // Палеонтологический журнал, 2018. № 2. С. 38–47.
214
Расницын А. П., Стрельникова О. Д. Дыхательная система и биология раннемелового ископаемого Saurophthirus longipes Ponomarenko, 1976 (Insecta, Aphaniptera, Saurophthiroidea stat. nov.) // Палеонтологический журнал, 2017. № 2. С. 61–72.
215
Witton M. P. Pterosaurs: Natural History, Evolution, Anatomy. Princeton: Princeton University Press, 2013. P. 164–169.
216
Щербаков Д. Е. Меловые Saurophthiridae (Aphaniptera) – куклородные протоблохи ныряющих птерозавров // Палеонтологический журнал, 2017. № 2. С. 73–75.
217
Шрётер Х. Сталинград. М.: Центрполиграф, 2004. С. 289.
218
Бержерак де С. С. Иной свет, или Государства и империи Луны // Новая Атлантида. – М.: Алгоритм, 2014. С. 116.
219
Cox R. et al.The ectoparasites of the European badger, Meles meles, and the behavior of the host-specific flea, Paraceras melis // Journal of Insect Behavior. 1999. Vol. 12. P. 245–265.
220
У представителей негроидной расы волосы плоские в поперечном сечении (отсюда их курчавость), тогда как у европейцев они округлые. Возможно, затруднения вшей в преодолении межрасового барьера связаны именно с этим. См.: Reed D. L. et al. Mammal hair diameter as a possible mechanism for host specialization in chewing lice // Journal of Mammalogy. 2000. Vol. 81. P. 999–1007.
221
Weiss R. A. Apes, lice and prehistory // Journal of Biology. 2009. Vol. 8: 20.
222
Arriaza B. et al. Severe Head Lice Infestation in an Andean Mummy of Arica, Chile // Journal of Parasitology. 2012. Vol. 98. P. 433–436.
223
Дубинин В. Б. Нахождение плейстоценовых вшей (Anoplura) и нематод при исследовании трупов индигирских ископаемых сусликов // Докл. АН СССР, 1948. Т. 62. № 3. С. 417–420.
224
Пухоедов делят на три подотряда – Amblycera, Ischnocera и Rhynchophthirina, причем, по молекулярным данным, первый из этих подотрядов может иметь независимое происхождение от двух остальных, которые дали начало сосущим вшам. См.: Johnson K. P. et al. Multiple origins of parasitism in lice // Proceedings of the Royal Society B. 2004. Vol. 271. P. 1771–1776.
225
Kingsolver J. G., Daniel T. L. Mechanics of food handling by fluid-feeding insects // Regulatory mechanisms in insect feeding (eds R. F. Chapman, G. de Boer), pp. 32–73. New York: Chapman & Hall, 1995. P. 32–73.
226
Rasnitsyn A. P., Zherikhin V. V. First fossil chewing louse from the Lower Cretaceous of Baissa, Transbaikalia (Insecta, Pediculida = Phthiraptera, Saurodectidae fam. n.) // Russian Entomological Journal. 1999. Vol. 8. P. 253–255.
227
Есть мнение, что эти «рога» следует интерпретировать не как выросты головной капсулы, а как базальные членики антенн, увеличенные в размерах. Антенны, модифицированные подобным образом, есть у самцов некоторых современных пухоедов из подотряда Ischnocera: они используются для удержания самок во время спаривания.
228
Gao T. et al. New insects feeding on dinosaur feathers in mid-Cretaceous amber // Nature Communications. 2019. Vol. 10: 5424. По другой версии, эти крошечные существа больше похожи на расселительную стадию червецов (Coccoidea). Подобно своим родичам тлям, червецы высасывают соки из растений и не имеют никакого отношения к эктопаразитизму. Во взрослом возрасте червецы малоподвижны, расселением у них занимаются личинки первого возраста, которых называют бродяжками. В поисках нужного растения бродяжки взбираются на все подряд – именно так они могли прицепиться к перьям, лежащим на земле. Интересно, что бродяжки тлей Adelgidae могут расселяться, влезая на оперение перелетных птиц. Grimaldi D.A., Vea I.M. Insects with 100 million-year-old dinosaur feathers are not ectoparasites // Nature Communications. 2021. Vol. 12: 1469.
229
Wappler T. et al. Scratching an Ancient Itch: An Eocene Bird Louse Fossil // Proceedings of the Royal Society of London B. 2004. Vol. 271. P. 255–258.
230
В гнездах можно встретить также сеноедов, относящихся к другим семействам, например Trogiidae.
231
Источник: https://en.wikibooks.org/wiki/Parasitic_Insects,_Mites_and_Ticks: _Genera_of_Medical_and_Veterinary_Importance; Lienhard C., Ashmole N. P. The Psocoptera (Insecta: Psocodea) of St Helena and Ascension Island (South Atlantic) with a new record from South Africa // Revue Suisse De Zoologie. 2011. Vol. 118. P. 423–449.
232
Azar D., Nel A. Evolution of hematophagy in «non-biting midges» (Diptera: Chironomidae) // Terrestrial Arthropod Reviews. 2012. Vol. 5. P. 15–34.
233
Hooke R. Micrographia, or, Some physiological descriptions of minute bodies made by magnifying glasses. L.: Martin J., Allestry J., 1665. P. 195.
234
Yang L. H., Gratton C. Insects as drivers of ecosystem processes // Current Opinion in Insect Science. 2014. Vol. 2. P. 26–32.
235
Batrons M. et al. Regional-Level Inputs of Emergent Aquatic Insects from Water to Land // Ecosystems. 2013. Vol. 16. P. 1353–1363.
236
McGinnis D. F. et al. Porewater methane transport within the gas vesicles of diurnally migrating Chaoborus spp.: An energetic advantage // Scientific Reports. 2017. Vol. 7: 44478.
237
Rumbos C. I. et al. Factors Affecting Leaf Litter Decomposition by Micropterna sequax (Trichoptera: Limnephilidae) // Hydrobiology. 2010. Vol. 95. P. 383–394.
238
Непокрытых А. В., Рожкова Н. А. Динамика количественных показателей популяции эндемичных ручейников (Trichoptera, Apataniidae) озера Байкал (Южный Байкал) // Вестн. БГСХА им. Филиппова, 2008. № 3. С. 59–65.
239
Johnson M. F. et al. Stabilization of fine gravels by net‐spinning caddisfly larvae // Earth Surface Processes and Landforms. 2009. Vol. 34. P. 413–423.
240
Wichard W. et al. The psychomyiid genus Palerasnitsynus (Insecta, Trichoptera) in mid-Cretaceous Burmese amber // Palaeodiversity. 2018. Vol. 11. P. 151–166.
241
Sinitshenkova N. D. Main ecological events in aquatic insects history // Acta zoologica cracoviensia. 2003. Vol. 46. P. 381–392.
242
Watson J. A. L., Theischinger G. The larva of Antipodophlebia asthenes (Tillyard): a terrestrial dragonfly? (Anisoptera: Aeshnidae) // Odonatologica. 1980. Vol. 9. P. 253–258.
243
Хотя личинки практически всех ныне живущих ручейников обитают в воде, некоторые из них (например, Enoicyla pusilla и Philocasca demita (Limnephilidae) приспособились к жизни во мху и листовом опаде. Стоит подчеркнуть, что все эти виды, так же как и современные стрекозы с сухопутными личинками, порвали с водной стихией недавно – их непосредственные предки на личиночной стадии жили в воде.
244
Жерихин В. В. Насекомые [озер позднего палеозоя и раннего мезозоя] // Избранные труды по палеоэкологии и филоценогенетике. – М.: КМК, 2003. – C. 121–125.
245
Woodruff C. D. Nuchal ligament reconstructions in diplodocid sauropods support horizontal neck feeding postures // Historical Biology. 2017. Vol. 29. P. 308–319.
246
Пономаренко А. Г. Членистоногие в эволюции континентальных водоемов // Вестник РАН, 2010. Т. 80. С. 880–889.
247
Калугина Н. С. Изменение подсемейственного состава хирономид (Diptera, Chironomidae) как показатель возможного эвтрофицирования водоемов в конце мезозоя // Бюллетень МОИП. Отд. биологии, 1974. Т. LXXIX (6). С. 45–56.
248
Львова А. А. и др. Роль донных организмов в трансформации органического вещества и в процессах самоочищения // Бентос Учинского водохранилища. – М.: Наука, 1980. – С. 171–176.
249
Nel A. et al. A new Upper Cretaceous species of Chresmoda from Lebanon – a latest representative of Chresmodidae (Insecta: Polyneoptera inc. sed.): first record of homeotic mutations in the fossil record of insects // European Journal of Entomology. 2004. Vol. 101. P. 145–151.
250
Bush J. W. M., Hu D. L. Walking on Water: Biolocomotion at the Interface // AnnualReview of Fluid Mechanics. 2006. Vol. 38. P. 339–361.
251
Andersen N. M. et al. A fossil Halobates from the Mediterranean and the originof sea skaters (Hemiptera, Gerridae) // Zoological Journal of the LinneanSociety. 1994. Vol. 112. P. 479–489.
252
Cheng L. Insects in marine environments // Marine insects / Cheng L. (ed). Amsterdam: North-Holland Publishing Company, 1976. P. 1–4.
253
Монтень М. Опыты. Кн. 1. М.: Изд-во АН СССР, 1954. С. 117.
254
Лютер М. О рабстве воли // Избранные произведения. СПб.: Андреев и согласие, 1994. С. 204.
255
Holter P. et al. Dung feeding in adult scarabaeines (tunnellers and endocoprids): even large dung beetles eat small particles // Ecological Entomology. 2002. Vol. 27. P. 169–176.
256
Фабр Ж.А. Инстинкт и нравы насекомых. Т. II. М.: Терра, 1993. С. 19.
257
Jacobs J. et al. First come, first serve: «sit and wait» behavior in dung beetles at the source of primate dung // Neotropical Entomology. 2008. Vol. 37. P. 641–645.
258
Doube B. M. Ecosystem services provided by dung beetles in Australia // Basic and Applied Ecology. 2018. Vol. 26. P. 35–49.
259
Bornemissza G. F. The Australian dung beetle project // Australian Meat Research Committee Review. 1976. Vol. 30. P. 1–30.
260
Sander P. M. et al. Biology of the sauropod dinosaurs: the evolution of gigantism // Biological Reviews. 2011. Vol. 86. P. 117–155.
261
Wilkinson D. M. et al. Could methane produced by sauropod dinosaurs have helped drive Mesozoic climate warmth? // Current Biology. 2012. Vol. 22. P. 292–293; Van Loon A. J. et al. Were sauropod dinosaurs responsible for the warm Mesozoic climate? // Journal of Palaeogeography. 2012. Vol. 1. P. 138–148.
262
Krell F. T. Fossil record and evolution of Scarabaeoidea (Coleoptera: Polyphaga) // Coleopterists Society Monograph. 2006. Vol. 5. P. 120–143.
263
Tarasov S. et al. A review and phylogeny of Scarabaeine dung beetle fossils (Coleoptera: Scarabaeidae: Scarabaeinae), with the description of two Canthochilum species from Dominican amber // Peer J. 2016. Vol. 4: e1988.
264
Arillo A., Ortuño V. M. Did dinosaurs have any relation with dung-beetles? (The origin of coprophagy) // Journal of Natural History. 2008. Vol. 42. P. 1405–1408.
265
Stavert J. R. et al. Dung beetles in an avian-dominated island ecosystem: feeding and trophic ecology // Oecologia. 2014. Vol. 176. P. 259–271.
266
Young O. P. The Attraction of Neotropical Scarabaeinae (Coleoptera: Scarabaeidae) to Reptile and Amphibian Fecal Material // The Coleopterists Bulletin. 1981. Vol. 35. P. 345–348.
267
Впрочем, стоит отметить, что в помете на птицефабриках разводятся в основном личинки мух Muscidae, а это семейство, как и другие калиптратные мухи, по эволюционным меркам очень молодо.
268
Vršanský P. et al. Cockroaches Probably Cleaned Up after Dinosaurs // PLoS ONE. 2013. Vol. 8: e80560.
269
Chin K., Gill B. D. Dinosaurs, Dung Beetles, and Conifers: Participants in a Cretaceous Food Web // Palaios. 1996. Vol. 11. P. 280–285.
270
Smith F. A. et al. The Evolution of Maximum Body Size of Terrestrial Mammals // Science. 2010. Vol. 330. P. 1216–1219.
271
Sánchez V. M. et al. Ichnostratigraphy of middle Cenozoic Coprinisphaera from central Patagonia: Insights into the evolution of dung beetles, herbivores and grass-dominated habitats // Palaeogeography, Palaeoclimatology, Palaeoecology. 2010. Vol. 297. P. 633–648.
272
Strömberg C. A. E. et al. Decoupling the spread of grasslands from the evolution of grazer-type herbivores in South America // Nature Communications. 2013. Vol. 4: 1478.
273
McAlpine J. F. First record of calypterate flies in the Mesozoic Era (Diptera: Calliphoridae) // The Canadian Entomologist. 1970. Vol. 102. P. 342–346.
274
Michelsen V. Oldest authentic record of a fossil calyptrate fly (Diptera): a species of Anthomyiidae from early Coenozoic Baltic amber // Studia Dipterologica. 2000. Vol. 7. P. 11–18.
275
Campobasso C. P. et al. Factors affecting decomposition and Diptera colonization // Forensic Science International. 2001. Vol. 120. P. 18–27.
276
Cai C.-Y. et al. Early origin of parental care in Mesozoic carrion beetles // Proceedings of the National Academy of Sciences. 2014. Vol. 111. P. 14170–14174.
277
Ingraham C. The animals that are most likely to kill you this summer // The Washington Post. 16.06.2015.
278
Лосский Н. О. Бог и мировое зло. М.: Республика, 1994. С. 359.
279
Бейтс Г. Натуралист на реке Амазонке. М.: Государственное издательство географической литературы, 1958.
280
Wilson E. O. The Case of the Social Insects // Excellence in Ecology. Vol. 2. Oldendorf/Luhe: Ecology Institute, 1990. P. 3–5.
281
Janzen D. H. Costa Rican Natural History. Chicago: The University of Chicago Press, 1983. P. 641.
282
Griffiths H. M. et al. Ants are the major agents of resource removal from tropical rainforests // Journal of Animal Ecology. 2018. Vol. 87. P. 293–300.
283
Самая разнообразная группа эусоциальных насекомых, муравьи (сем. Formicidae), насчитывает всего 15 000 видов и подвидов – гораздо меньше, чем многие семейства жуков и наездников.
284
Edgerly J. S. Maternal behaviour of a webspinner (Order Embiidina) // Ecological Entomology. 1987. Vol. 12. P. 1–11.
285
На этот счет биолог Уильям Гамильтон сформулировал правило, которому позже было присвоено его имя. В математической форме оно записывается как rB > C, где r – коэффициент родства, B – вклад в чужой репродуктивный успех, С – издержки, которые организм понес, помогая другому выжить и размножиться (обычно они выражаются в недопроизводстве собственного потомства). Соответственно, расходы, связанные с альтруистическим поведением особи, должны окупаться за счет косвенного вклада в распространение ее генов, однако в случае низкого коэффициента родства этот вклад будет слишком мал, чтобы их окупить.
286
По-другому ее называют теорией охватывающей приспособленности (inclusive fitness).
287
Докинз Р. Эгоистичный ген. – М.: АСТ, CORPUS, 2013.
288
Позднее Уилсон пересмотрел свои взгляды и выступил с критикой теории родственного отбора, после чего более 100 биологов написали коллективное письмо в ее защиту. Эта полемика произошла на страницах журнала Nature в 2011 г. См., в частности: Abbot P. et al. Inclusive fitness theory and eusociality // Nature. 2011. Vol. 471. P. 1–4.
289
Расницын А. П. Происхождение социальности муравьев // Избранные труды по эволюционной биологии. – М.: КМК, 2005. – С. 260–268.
290
Wilson E. O. Sociobiology: The New Synthesis. Bridge: Belknap Press of Harvard University Press, 1975.
291
Peer K., Taborskyi M. Outbreeding depression, but no inbreeding depression in haplodiploid ambrosia beetles with regular sibling mating // Evolution. 2005. Vol. 59. P. 317–323.
292
Mound L. A., Crespi B. J. Biosystematics of two new gall-inducing thrips with soldiers (Insecta: Thysanoptera) from Acacia trees in Australia // Journal of Natural History. 1995. Vol. 29. P. 147–157. Сформировавшиеся яйца ученые обнаружили только у 7–12 % всех самок-солдат Oncothrips tepperi и O. habrus. У другого вида эусоциальных трипсов, Kladothrips hamiltoni, самки-солдаты вносят существенно больший вклад в размножение: их потомство составляет 60–80 % расселительного поколения, которое производит колония за сезон. Тем не менее каждая самка-солдат в среднем откладывает в три раза меньше яиц, чем ее мать – самка-основательница. Примечательно, что самки-солдаты вынуждены спариваться со своими братьями-солдатами, т. е. инцест играет в жизни эусоциальных трипсов не меньшую роль, чем у амброзиевых жуков или голых землекопов. Kranz B. D. et al. Social biology and sex ratios of the eusocial gall-inducing thrips Kladothrips hamiltoni // Ecological Entomology. 1999. Vol. 24. P. 432–442.
293
Kurosu U., Shigeyuki A. Extremely Long-Closed Galls of a Social Aphid // Psyche. 2009. Vol. 2009: 159478.
294
Kutsukake M. et al. Plant Manipulation by Gall-Forming Social Aphids for Waste Management // Frontiers in Plant Science. 2019. Vol. 10: 933.
295
Benton T. G., Foster W. A. Altruistic Housekeeping in a Social Aphid // Proceedings of the Royal Society B: Biological Sciences. 1992. Vol. 247. P. 199–202.
296
Reeve H. K. et al. DNA “fingerprinting” reveals high levels of inbreeding in colonies of the eusocial naked mole-rat // Proceedings of the National Academy of Sciences. 1990. Vol. 87. P. 2496–2500.
297
Duffy J. E. Eusociality in a coral-reef shrimp // Nature. 1996. Vol. 381. P. 512–514.
298
Интересно, что некоторые паразитоидные перепончатокрылые все же демонстрируют элементы эусоциального поведения, как, например, наездник Copidosoma floridanum (Encyrtidae). Самка этого вида откладывает в свою жертву, гусеницу, одно-единственное яйцо, дающее начало целой армии однояйцевых близнецов-клонов, возникающих за счет многократного деления исходного эмбриона. В итоге внутри гусеницы формируется настоящая паразитоидная колония общей численностью до 3000 личинок. Но не всем из них суждено превратиться во взрослых ос. До четверти личинок прерывают развитие и становятся солдатами, жертвуя своим будущим во имя безопасности братьев или сестер. Как уже отмечалось (см. главу 7), между паразитоидами идет жесткая конкуренция за подходящую жертву, что порой приводит к гиперпаразитизму. Личинки-солдаты нужны для предотвращения этой опасности. Если другая оса посмеет отложить свое яйцо в гусеницу, где хозяйничает паразитоидная колония, то личинки-солдаты накинутся на личинку-чужака и убьют ее. Но, несмотря на наличие бесплодной касты солдат, о полноценной эусоциальности у полиэмбриональных наездников говорить не приходится, ведь в рамках личиночных колоний не сосуществуют разные поколения и нет совместного ухода за потомством. См.: Giron D. et al. Male soldier caste larvae are non-aggressive in the polyembryonic wasp Copidosoma floridanum // Biology Letters. 2007. Vol. 3. P. 431–434.
299
West-Eberhard M. J. Behavior of the primitively social wasp Montezumia cortesioides Willink (Vespidae: Eumeninae) and the origins of vespid sociality // Ethology Ecology & Evolution. 2005. Vol. 17. P. 201–215.
300
Таких муравьев-рабочих, перешедших к размножению, называют гамергатами.
301
Brockmann H. J. Cooperative breeding in wasps and vertebrates: the role of ecological constraints // The evolution of social behaviour in insects and arachnids / J. C. Choe & B. J. Crespi (eds.). Cambridge: Cambridge University Press, 1997. P. 347–371.
302
Walters J. R. et al. Test of the Ecological Basis of Cooperative Breeding in Red-Cockaded Woodpeckers // The Auk. 1992. Vol. 109. P. 90–97.
303
Linsenmair K. E. Individual and family recognition in subsocial arthropods, in particular in the desert isopod Hemilepistus reaumuri // Fortschritte der Zoologie. Bd. 31. Experimental Behavioral Ecology and Sociobiology. Stuttgart: G. Fisher Verl, 1985. P. 411–436.
304
Schuster J. C., Schuster L. B. Social Behavior in Passalid Beetles (Coleoptera: Passalidae): Cooperative Brood Care // The Florida Entomologist. 1985. Vol. 68. P. 266–272.
305
Hamilton W. D. The Genetical Evolution of Social Behaviour. I, II // Journal of Theoretical Biology. 1964. Vol. 7. P. 1–16, 17–52.
306
Baer B. et al. Sperm storage induces an immunity cost in ants // Nature. 2006. Vol. 441. P. 872–875; Dávila F. et al. Antibacterial activity of male and female sperm-storage organs in ants // Journal of Experimental Biology. 2018. Vol. 221: jeb175158.
307
Boomsma J. J., Ratnieks F. L. W. Paternity in eusocial Hymenoptera // Philosophical Transactions of the Royal Society B. 1996. Vol. 351. P. 947–975.
308
Boomsma J. J. Lifetime monogamy and the evolution of eusociality // Philosophical Transactions of the Royal Society B. 2009. Vol. 364. P. 3191–3207; Cornwallis C. K. et al. Promiscuity and the evolutionary transition to complex societies // Nature. 2010. Vol. 466. P. 969–972; Hughes W. O.H. et al. Ancestral monogamy shows kin selection is key to the evolution of eusociality // Science. 2008. Vol. 320. P. 1213–1216.
309
Эусоциальность голых землекопов также была предсказана. Это произошло в 1975–1976 гг., когда эусоциальные виды были известны только среди насекомых. Автор предсказания, американский биолог Ричард Александер, пришел к выводу, что эусоциальные млекопитающие, подобно термитам, могли бы жить в подземных ходах и питаться клубнями растений, не вылезая на поверхность. Свои идеи на этот счет ученый изложил в ряде лекций и семинаров. На одном из них кто-то заметил, что гипотетические существа, описываемые Александером, хорошо соответствуют африканским голым землекопам. Заинтригованный, Александер связался c Дженнифер Джервис из ЮАР, которая занималась этими грызунами. На тот момент о социальной жизни голых землекопов было ничего не известно, но Джервис стала работать в этом направлении, результатом чего стала ее статья в Science в 1981 г., подтвердившая справедливость догадки Александера. См.: Jarvis J. U. Eusociality in a mammal: cooperative breeding in naked mole-rat colonies // Science. 1981. Vol. 212. P. 571–573.
310
В виде исключения гапло-диплоидия на уровне отдельных родов и семейств встречается также среди жуков и равнокрылых. У трипсов же и перепончатокрылых это характеристика всего отряда – других таких отрядов в системе насекомых нет. См.: Mable B. K., Otto S. P. The evolution of life cycles with haploid and diploid phases // BioEssays. 1998. Vol. 20. P. 453–462.
311
Дарвин Ч. Происхождение человека и половой отбор // Сочинения. Т. 5. М.: АН СССР, 1953. С. 177.
312
Strausfeld N. Arthropod Brains: Evolution, Functional Elegance, and Historical Significance. London: The Belknap Press of Harvard University Press, 2012. P. 237.
313
Strausfeld N. J. et al. Evolution, Discovery, and Interpretations of Arthropod Mushroom Bodies // Learning Memory. 1998. Vol. 5. P. 11–37.
314
Strausfeld N. Arthropod Brains: Evolution, Functional Elegance, and Historical Significance. London: The Belknap Press of Harvard Uni-versity Press, 2012. P. 257.
315
Farris S. M., Schulmeister S. Parasitoidism, not sociality, is associated with the evolution of elaborate mushroom bodies in the brains of hymenopteran insects // Proceedings of the Royal Society B. 2011. Vol. 278. P. 940–951.
316
Stern D. L., Foster W. A. The evolution of soldiers in aphids // Biological Reviews. 1996. Vol. 71. P. 27–79.
317
Kutsukake M. et al. Exaggeration and cooption of innate immunity for social defense // Proceedings of the National Academy of Sciences. 2019. Vol. 116. P. 8950–8959.
318
Crespi B. J. Eusociality in Australian gall-thrips // Nature. 1992. Vol. 359. P. 724–726.
319
Park Y., Choe J. Territorial behavior of the Korean wood-feeding cockroach, Cryptocercus kyebangensis // Journal of Ethology. 2003. Vol. 21. P. 79–85.
320
У тех видов термитов, у которых каста солдат отсутствует, это считается результатом вторичной утраты.
321
Engel M. S. et al. Morphologically Specialized Termite Castes and Advanced Sociality in the Early Cretaceous // Current Biology. 2016. Vol. 26. P. 522–530.
322
Ориген. О началах. Против Цельса. СПб.: Библиополис, 2008. С. 768.
323
Cunningham J.P. et al. Bees at War: Interspecific Battles and Nest Usurpation in Stingless Bees // The American Naturalist. 2014. Vol. 184. P. 777–786.
324
Barden P., Grimaldi D. A. Adaptive Radiation in Socially Advanced Stem-Group Ants from the Cretaceous // Current Biology. 2016. Vol. 26. P. 515–521.
325
Meyer C. et al. The massacre mass grave of Schöneck-Kilianstädten reveals new insights into collective violence in Early Neolithic Central Europe // Proceedings of the National Academy of Sciences. 2015. Vol. 112. P. 11217–11222.
326
Deligne J. et al. The Enemies and Defense Mechanisms of Termites // Social insects. Vol. II / Hermann H. R. (ed). New York: Academic Press, 1981. P. 1–76; Prestwich G. D. Defense mechanisms of termites // Annual Review of Entomology. 1984. Vol. 29. P. 201–232; Šobotník J. et al. Explosive Backpacks in Old Termite Workers // Science. Vol. 337. P. 436.
327
Engel M. S. et al. Morphologically Specialized Termite Castes and Advanced Sociality in the Early Cretaceous // Current Biology. 2016. Vol. 26. P. 522–530.
328
Higashi S., Ito F. Defense of termitaria by termitophilous ants // Oecologia. 1989. Vol. 80. P. 145–147.
329
Coty D. et al. The First Ant-Termite Syninclusion in Amber with CT-Scan Analysis of Taphonomy // PLoS ONE. 2014. Vol. 9: e104410.
330
Гексли Т. Г. О положении человека в ряду органических существ. – СПб.: Н. Таблен, 1864.
331
К одиночному образу жизни пчел подталкивает суровый холодный климат. См.: Eickwort G. C. et al. Solitary behavior in a high altitude population of the social sweat bee Halictus rubicundus (Hymenoptera: Halictidae) // Behavioral Ecology and Sociobiology. 1996. Vol. 38. P. 227–233. Интересно, что даже члены одной популяции в зависимости от времени года также могут становиться одиночками либо жить в коллективе. См.: Yanega D. Social plasticity and early-diapausing females in a primitively social bee // Proceedings of the National Academy of Sciences. 1988. Vol. 85. P. 4374–4377
332
С эволюционной точки зрения пчелы – потомки хищных сфекоидных ос, которые вместо умерщвленных членистоногих стали заготовлять своим личинкам пыльцу, для чего обзавелись рядом адаптаций, таких как ветвистые волоски и расширенный первый членик задней лапки. К кормлению личинок пыльцой независимо от пчел перешли и некоторые другие жалящие перепончатокрылые, такие как, например, цветочные осы (Masarinae) из семейства складчатокрылых ос.
333
Ross K. G., Matthews R. W. New evidence for eusociality in the sphecid wasp Microstigmus comes // Animal Behaviour. 1989. Vol. 38. P. 613–619.
334
В качестве единственного известного мне исключения могу назвать россыпь окаменевших муравьев, их куколок и личинок, найденную в нижнем миоцене Кении и представляющую собой остатки одного крупного гнезда. Впрочем, об архитектуре этого гнезда данных нет – возможно, муравьиные ходы были разрушены в процессе раскопок. См.: Wilson E. O., Taylor R. W. A Fossil Ant Colony: New Evidence of Social Antiquity // Psyche: A Journal of Entomology. 1964. Vol. 71. P. 93–103.
335
Wenzel J. W. A social wasp’s nest from the Cretaceous period, Utah, USA, and its biographical significance // Psyche. 1990. Vol. 97. P. 21–29.
336
Не считая, разумеется, крылатых самок и самцов, которые вылетают из муравейников в период размножения.
337
Например, бескрылых самок можно встретить среди семейств Bethylidae, Bradynobaenidae, Mutillidae и Tiphiidae. При этом у видов с бескрылыми самками самцы остаются крылатыми.
338
Коленчатые антенны – не такой надежный отличительный признак муравьев, как отверстия метаплевральных желез, поскольку изредка антенны подобного строения встречаются и у одиночных ос, как, например, у бескрылых ос Aptenoperissidae, найденных в бирманском янтаре.
339
Длусский Г. М., Расницын А. П. Палеонтологическая летопись и этапы эволюции муравьев // Успехи современной биологии, 2007. Т. 127. С. 118–134.
340
Wilson E. O. The earliest known ants: an analysis of the Cretaceous species and an inference concerning their social organization // Paleobiology. 1987. Vol. 13. P. 44–53.
341
Barden P. et al. Specialized predation drives aberrant morphological integration and diversity in the earliest ants // Current Biology. 2020. Vol. 30. P. 3818–3824.e4.
342
Barden P. Fossil ants (Hymenoptera: Formicidae): ancient diversity and the rise of modern lineages // Myrmecological News. 2017. Vol. 24. P. 1–30.
343
Wilson E. O. 1990. Success and Dominance in Ecosystems: The Case of the Social Insects. Oldendorf/Luhe (Germany): Ecology Institute. 104 pp.
344
Из них наиболее разнообразными и массовыми являются подсемейства Dolichoderinae, Formicinae, Myrmicinae и Ponerinae.
345
LaPolla J. S. et al. Ants and the Fossil Record // Annual Review of Entomology. 2013. Vol. 609–630.
346
Это наблюдается далеко не у всех насекомых – например, тли, шмели и наездники-ихневмониды гораздо разнообразнее в умеренном климате, чем в тропиках.
347
Не путать с ныне живущим подсемейством Formicinae, к которому относятся, например, рыжие лесные муравьи.
348
Archibald B. S. Intercontinental dispersal of giant thermophilic ants across the Arctic during early Eocene hyperthermals // Proceedings of the Royal Society B: Biological Sciences. Vol. 278. P. 3679–3686.
349
Dorn R. I. Ants as a powerful biotic agent of olivine and plagioclase dissolution // Geology. 2014. Vol. 42. P. 771–774.
350
Lyford W. H. Importance of ants to brown podzolic soil genesis in New England // Harvard Forest Paper. 1963. № 7. P. 1–18.
351
Bull J. W., Maron M. How humans drive speciation as well as extinction // Proceedings of the Royal Society B. 2016. Vol. 283: 20160600.
352
Мирмекофилы существуют и среди птиц. К ним относятся американские муравьеловки (Thamnophilidae), которые следуют за колоннами кочевых муравьев и ловят насекомых, вспугнутых их приближением (вопреки своему русскому названию, самими муравьями эти птицы не питаются). Некоторые муравьеловки способны находить себе пропитание и независимо от муравьев, однако 16 видов птиц этого семейства разучились питаться самостоятельно и полагаются исключительно на их содействие. См.: Brumfield R. T. et al. Phylogenetic conservatism and antiquity of a tropical specialization: Army-ant-following in the typical antbirds (Thamnophilidae) // Molecular Phylogenetics and Evolution. 2007. Vol. 45. P. 1–13.
353
Parker J. Myrmecophily in beetles (Coleoptera): evolutionary patterns and biological mechanisms // Myrmecological News. 2016. Vol. 22:65–108.
354
Hölldobler B. et al. Behavior and exocrine glands in the myrmecophilous beetle Lomechusoides strumosus (Fabricius, 1775) (formerly called Lomechusa strumosa) (Coleoptera: Staphylinidae: Aleocharinae) // PLoS ONE. 2018. Vol. 13: e0200309.
355
Parker J., Grimaldi D. A. Specialized Myrmecophily at the Ecological Dawn of Modern Ants // Current Biology. 2014. Vol. 24. P. 2428–2434.
356
Maderspacher F., Stensmyr M. Myrmecomorphomania // Current Biology. 2011. Vol. 21. P. R291–R293.
357
Oliver T. H. et al. Macroevolutionary patterns in the origin of mutualisms involving ants // Journal of Evolutionary Biology. 2008. Vol. 21. P. 1597–1608.
358
Зобик – это расширенный карман передней кишки муравьев. Именно в зобике хранится та питательная жидкость, которой муравьи обмениваются при трофаллаксисе.
359
Johnson C. D. et al. Acropyga and Azteca ants (Hymenoptera: Formicidae) with scale insects (Sternorrhyncha: Coccoidea): 20 million years of intimate symbiosis // American Museum Novitates. 2001. Vol. 3335. P. 1–18.
360
Перковский Е. Э. Сининклюзы муравьев Lasius schiefferdeckeri (Hymenoptera, Formicidae) и тлей рода Germaraphis (Homoptera, Aphidinea) в ровенском и саксонском янтаре // Вестн. зоол., 2007. Т. 41. № 2. С. 181–185. Перковский Е. Э. Распределение тлей рода Germaraphis (Homoptera, Aphidinea) в ровенских янтарях различного веса и их сининклюзы с муравьями // Вестн. зоол., 2010. Т. 44. № 1. С. 55–62.
361
Изменением состава растительности это массовое вымирание тлей на рубеже мела и палеогена объяснить нельзя, поскольку экспансия цветковых произошла значительно раньше. См.: Perkovsky E., Wegierek P. Aphid – Buchnera – Ant symbiosis; or why are aphids rare in the tropics and very rare further south? // Earth and Environmental Science Transactions of The Royal Society of Edinburgh. Vol. 107. P. 297–310.
362
Pemberton R. W. Fossil Extrafloral Nectaries, Evidence for the Ant-Guard Antiherbivore Defense in an Oligocene Populus // American Journal of Botany. 1992. Vol. 79. P. 1242–1246.
363
Rico-Gray V., Oliveira P. S. The ecology and evolution of ant-plant interactions. Chicago: University of Chicago Press, 2007. 320 pp.
364
Lengyel S. et al. Ants Sow the Seeds of Global Diversification in Flowering Plants // PLoS ONE. 2009. Vol. 4(5): e5480.
365
Gao T. et al. Maternal care by Early Cretaceous cockroaches // Journal of Systematic Palaeontology. 2019. Vol. 17. P. 379–391.
366
Seelinger G., Seelinger U. On the Social Organisation, Alarm and Fighting in the Primitive Cockroach Cryptocercus punctulatus Scudder // Zeitschrift für Tierpsychologie. 1983. Vol. 61. P. 315–333.
367
Nalepa C. A. Colony composition, protozoan transfer and some life history characteristics of the woodroach Cryptocercus punctulatus Scudder (Dictyoptera: Cryptocercidae) // Behavioral Ecology and Sociobiology. 1984. Vol. 14. P. 273–279.
368
Nalepa C. A., Lenz M. The ootheca of Mastotermes darwiniensis Froggatt (Isoptera: Mastotermitidae): homology with cockroach oothecae // Proceedings of the Royal Society B: Biological Sciences. 2000. Vol. 267. P. 1809–1813.
369
Nalepa C. A. Altricial Development in Wood-Feeding Cockroaches: The Key Antecedent of Termite Eusociality // Biology of Termites: a Modern Synthesis / D. E. Bignell, Y. Roisin, N. Lo (eds). Springer: Dordrecht, 2011. P. 69–95.
370
Farris S. M., Strausfeld N. J. A Unique Mushroom Body Substructure Common to Basal Cockroaches and to Termites // Journal of Comparative Neurology. 2003. Vol. 456. P. 305–320.
371
А вот лигнин термиты расщеплять не могут. С этой задачей справляются только термиты-фермеры с помощью грибов.
372
Govorushko S. Economic and ecological importance of termites: A global review. Entomological Science. 2019. Vol. 22. P. 21–35.
373
Wier et al. Spirochete and protist symbionts of a termite (Mastotermes electrodominicus) in Miocene amber // Proceedings of the National Academy of Sciences. 2002. Vol. 99. P. 1410–1413.
374
Slaytor M. Сellulose digestion in termites and cockroaches: What role do symbionts play? // Comparative Biochemistry and Physiology Part B. 1992. Vol. 103. P. 775–784.
375
Hongoh Y. et al. Genome of an Endosymbiont Coupling N>2 Fixation to Cellulolysis Within Protist Cells in Termite Gut // Science. 2008. Vol. 322. P. 1108–1109.
376
Bignell D. E. Termites as Soil Engineers and Soil Processors // Intestinal Microorganisms of Termites and other Invertebrates / Koenig, H., Varma A. (eds.) Springer: Berlin, 2006. P. 183–220.
377
Nalepa C. Nourishment and the origin of termite eusociality // Nourishment and Evolution in Insect Societies / Hunt J. H., Nalepa C., (eds.) Boulder: Westview Press; 1994. P. 57–104
378
Nalepa C. A. What Kills the Hindgut Flagellates of Lower Termites during the Host Molting Cycle? // Microorganisms. 2017. Vol. 5:82.
379
Для этого вида известна лишь крылатая особь, вылетевшая из термитника для размножения и угодившая в древний водоем. Бескрылые термиты-рабочие, которые всю жизнь проводят под землей или в трухлявой древесине, падают в водоемы гораздо реже и потому в виде каменных отпечатков почти не встречаются. Практически все, что мы знаем о древних термитах-рабочих, ограничивается янтарными находками. Это же, кстати, относится и к муравьям.
380
Engel M. S. et al. Termites (Isoptera): Their Phylogeny, Classification, and Rise to Ecological Dominance // American Museum Novitates. 2009. Vol. 3650. P. 1–27.
381
Надо сказать, что и у низших термитов функциональная нагрузка лежит не на самих жгутиконосцах, а на бактериях, расположенных внутри них или же на их клеточной мембране. Не совсем ясно, в чем заключается смысл такого посредничества и почему низшие термиты, как и высшие, не могут использовать бактерий напрямую.
382
Perkovsky E. E., Vasilenko D. V. Evolution of tropical termites in early Paleogene with description of a new species of Stylotermitidae (Isoptera) from Rovno amber (late Eocene of Ukraine) // Invertebrate Zoology. 2020. Vol. 17. P. 231–246. Согласно другой гипотезе, все произошло наоборот: сначала термиты начали питаться землей, и только потом у них из кишечника пропали жгутиконосцы, не выдержавшие этого изменения в рационе своих хозяев. См.: Chouvenc T. et al. Termite evolution: mutualistic associations, key innovations, and the rise of Termitidae // Cellular and Molecular Life Sciences. 2021. Vol. 78. P. 2749–2769.
383
Rohr D. M. et al. Oldest termite nest from the Upper Cretaceous of west Texas (USA) // Geology. 1986. Vol. 14. P. 87–88.
384
Bignell D. E. Op. cit.
385
Martin S.J. et al. A vast 4,000-year-old spatial pattern of termite mounds // Current Biology. 2018. Vol. 28. P. R1292–R1293.
386
Sugimoto A. et al. Global impact of termites on the carbon cycle and atmospheric trace gases // Termites: evolution sociality, symbiosis, ecology / Abe T., Bignell D. E., Higashi M. (eds). Dordrecht: Kluwer Academic Publishers. P. 409–435.
387
Kistner D. H. The biology of termitophiles // Biology of Termites / K. Krishna, F. M. Weesner (eds.), New York: Academic Press, 1969. Vol. 1. P. 525–557.
388
Cai et al. Early evolution of specialized termitophily in Cretaceous rove beetles // Current Biology. 2017. Vol. 27. P. 1229–1235.
389
Johnson J.B., Hagen K. S. A neuropterous larva uses an allomone to attack termites // Nature. 1981. Vol. 289. P. 506–507.
390
Mueller U. G. et al. The Evolution of Agriculture in Insects // Annual Review of Ecology Evolution and Systematics. 2005. Vol. 36. P. 563–595.
391
Lincecum G. Notice on the Habits of the «Agricultural Ant» of Texas [ «Stinging Ant» or «Mound-making Ant» Myrmica (Atta) malefaciens, Buckley] // Zoological Journal of the Linnean Society. 1861. Vol. 6. P. 29–31.
392
Chomicki G., Renner S. S. Obligate plant farming by a specialized ant // Nature Plants. 2016. 2: 16181.
393
Belt T. The Naturalist in Nicaragua. L.: J. Murray, 1874.
394
Wilson E. O. Caste and division of labor in leaf-cutter ants (Hymenoptera: Formicidae: Atta) // Behavioral Ecology and Sociobiology. 1980. Vol. 7. P. 143–156.
395
Jonkman J. C. Average vegetative requirement, colony size and estimated impact of Atta vollenweideri on cattle‐raising in Paraguay // Zeitschrift für Angewandte Entomologie. 1980. Vol. 89. P. 135–143.
396
Costa A. N. et al. Do herbivores exert top‐down effects in Neotropical savannas? Estimates of biomass consumption by leaf‐cutter ants // Journal of Vegetation Science. 2008. Vol. 19. P. 849–854.
397
Herz H. et al. Herbivory Rate of Leaf‐Cutting Ants in a Tropical Moist Forest in Panama at the Population and Ecosystem Scales // Biotropica. 2007. Vol. 39. P. 482–488.
398
Nygaard S. et al. Reciprocal genomic evolution in the ant – fungus agricultural symbiosis // Nature Communications. 2016. Vol. 7: 12233.
399
Genise J. F. et al. Attaichnus kuenzelii revisited: A Miocene record of fungus-growing ants from Argentina // Palaeogeography, Palaeoclimatology, Palaeoecology. 2013. Vol. 386. P. 349–363.
400
Roberts E.M. et al. Oligocene Termite Nests with In Situ Fungus Gardens from the Rukwa Rift Basin, Tanzania, Support a Paleogene African Origin for Insect Agriculture // PLoS ONE. 2016. Vol. 11: e0156847.
401
Branstetter M. G. et al. Dry habitats were crucibles of domestication in the evolution of agriculture in ants. Proceedings of the Royal Society B. Vol. 284: 20170095; Aanen D. K., Eggleton P. Fungus-Growing Termites Originated in African Rain Forest // Current Biology. 2005. Vol. 15. P. 851–855.
402
См.: Nobre T. et al. Comparative Biology of Fungus Cultivation in Termites and Ants // Biology of Termites: a Modern Synthesis / D. E. Bignell, Y. Roisin, N. Lo (eds). Springer: Dordrecht, 2011. P. 193–210.
403
Aaren D. K. As you reap, so shall you sow: coupling of harvesting and inoculating stabilizes the mutualism between termites and fungi // Biology Letters. 2006. Vol. 2. P. 209–212.
404
К гликозил-гидролазам относятся, в частности, различные целлюлазы и пектиназы, о которых мы уже говорили.
405
Poulsen M. et al. Complementary symbiont contributions to plant decomposition in a fungus-farming termite // Proceedings of the National Academy of Sciences. 2014. Vol. 111. P. 14500–14505.
406
Poinar G. O. et al. New subfamily of ambrosia beetles (Coleoptera: Platypodidae) from mid-Cretaceous Burmese amber // Historical Biology. 2020. Vol. 32. P. 137–142.
407
Biedermann P. H. W., Taborsky M. Larval helpers and age polyethism in ambrosia beetles // Proceedings of the National Academy of Sciences. 2011. Vol. 108. P. 17064–17069.
408
Sheikh I. U. Utilization of silkworm pupae meal as an alternative source of protein in the diet of livestock and poultry: A review // Journal of Entomology and Zoology Studies. 2018. Vol. 6. P. 1010–1016.
409
Layard A. Discoveries in the Ruins of Nineveh and Babylon. London: John Murray, 1853. P. 339.
410
Судя по описанию Давида Ливингстона в его «Путешествиях и исследованиях миссионера в Южной Африке», технология добычи термитов в XIX в. не слишком отличалась от той, что использовали австралопитеки. По словам Ливингстона, туземцы сначала пробивают дыру в термитнике, ждут какое-то время рядом и затем смахивают в сосуд термитов-рабочих, вылезших, чтобы ее заделать.
411
Backwell D. R., d’Errico F. First evidence of termite-foraging by Swartkrans early hominids // Proceedings of the National Academy of Sciences. 2001. Vol. 98. P. 1358–1363.
412
Modesto S. P. et al. Arthropod remains in the oral cavities of fossil reptiles support inference of early insectivory // Biology Letters. 2009. Vol. 5. P. 838–840.
413
Sues H.-D., Reisz R. R. Origins and early evolution of herbivory in tetrapods // Trends in Ecology & Evolution. 1998. Vol. 13. P. 141–145.
414
Qvarnström M. et al. 2019. Beetle-bearing coprolites possibly reveal the diet of a Late Triassic dinosauriform // Royal Society Open Science, 2019, 6: 181042.
415
Rayfield E. J. Dietary specializations and diversity in feeding ecology of the earliest stem mammals // Nature. 2014. Vol. 512. P. 303–305.
416
Payne C. L. R. Are edible insects more or less ‘healthy’ than commonly consumed meats? A comparison using two nutrient profiling models developed to combat over– and undernutrition // European Journal of Clinical Nutrition. 2016. Vol. 70. P. 285–291.
417
Johnson C. K. et al. Global shifts in mammalian population trends reveal key predictors of virus spillover risk // Proceedings of the Royal Society B. 2020. Vol. 287: 20192736.
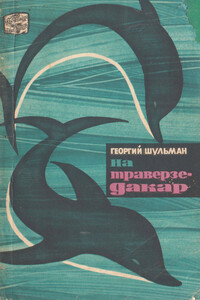
Послевоенные годы знаменуются решительным наступлением нашего морского рыболовства на открытые, ранее не охваченные промыслом районы Мирового океана. Одним из таких районов стала тропическая Атлантика, прилегающая к берегам Северо-западной Африки, где советские рыбаки в 1958 году впервые подняли свои вымпелы и с успехом приступили к новому для них промыслу замечательной деликатесной рыбы сардины. Но это было не простым делом и потребовало не только напряженного труда рыбаков, но и больших исследований ученых-специалистов.
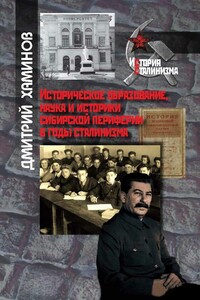
Настоящая монография посвящена изучению системы исторического образования и исторической науки в рамках сибирского научно-образовательного комплекса второй половины 1920-х – первой половины 1950-х гг. Период сталинизма в истории нашей страны характеризуется определенной дихотомией. С одной стороны, это время диктатуры коммунистической партии во всех сферах жизни советского общества, политических репрессий и идеологических кампаний. С другой стороны, именно в эти годы были заложены базовые институциональные основы развития исторического образования, исторической науки, принципов взаимоотношения исторического сообщества с государством, которые определили это развитие на десятилетия вперед, в том числе сохранившись во многих чертах и до сегодняшнего времени.
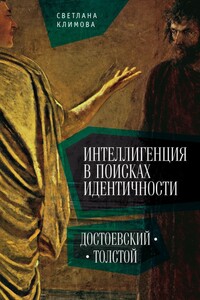
Монография посвящена проблеме самоидентификации русской интеллигенции, рассмотренной в историко-философском и историко-культурном срезах. Логически текст состоит из двух частей. В первой рассмотрено становление интеллигенции, начиная с XVIII века и по сегодняшний день, дана проблематизация важнейших тем и идей; вторая раскрывает своеобразную интеллектуальную, духовную, жизненную оппозицию Ф. М. Достоевского и Л. Н. Толстого по отношению к истории, статусу и судьбе русской интеллигенции. Оба писателя, будучи людьми диаметрально противоположных мировоззренческих взглядов, оказались “versus” интеллигентских приемов мышления, идеологии, базовых ценностей и моделей поведения.
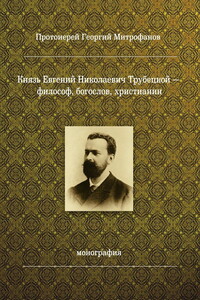
Монография протоиерея Георгия Митрофанова, известного историка, доктора богословия, кандидата философских наук, заведующего кафедрой церковной истории Санкт-Петербургской духовной академии, написана на основе кандидатской диссертации автора «Творчество Е. Н. Трубецкого как опыт философского обоснования религиозного мировоззрения» (2008) и посвящена творчеству в области религиозной философии выдающегося отечественного мыслителя князя Евгения Николаевича Трубецкого (1863-1920). В монографии показано, что Е.
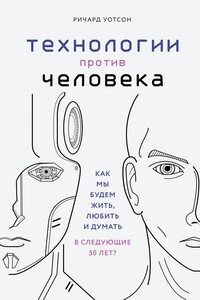
Эксперты пророчат, что следующие 50 лет будут определяться взаимоотношениями людей и технологий. Грядущие изобретения, несомненно, изменят нашу жизнь, вопрос состоит в том, до какой степени? Чего мы ждем от новых технологий и что хотим получить с их помощью? Как они изменят сферу медиа, экономику, здравоохранение, образование и нашу повседневную жизнь в целом? Ричард Уотсон призывает задуматься о современном обществе и представить, какой мир мы хотим создать в будущем. Он доступно и интересно исследует возможное влияние технологий на все сферы нашей жизни.
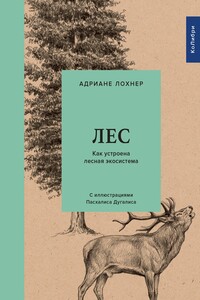
Что такое, в сущности, лес, откуда у людей с ним такая тесная связь? Для человека это не просто источник сырья или зеленый фитнес-центр – лес может стать местом духовных исканий, служить исцелению и просвещению. Биолог, эколог и журналист Адриане Лохнер рассматривает лес с культурно-исторической и с научной точек зрения. Вы узнаете, как устроена лесная экосистема, познакомитесь с различными типами леса, характеризующимися по составу видов деревьев и по условиям окружающей среды, а также с видами лесопользования и с некоторыми аспектами охраны лесов. «Когда видишь зеленые вершины холмов, которые волнами катятся до горизонта, вдруг охватывает оптимизм.